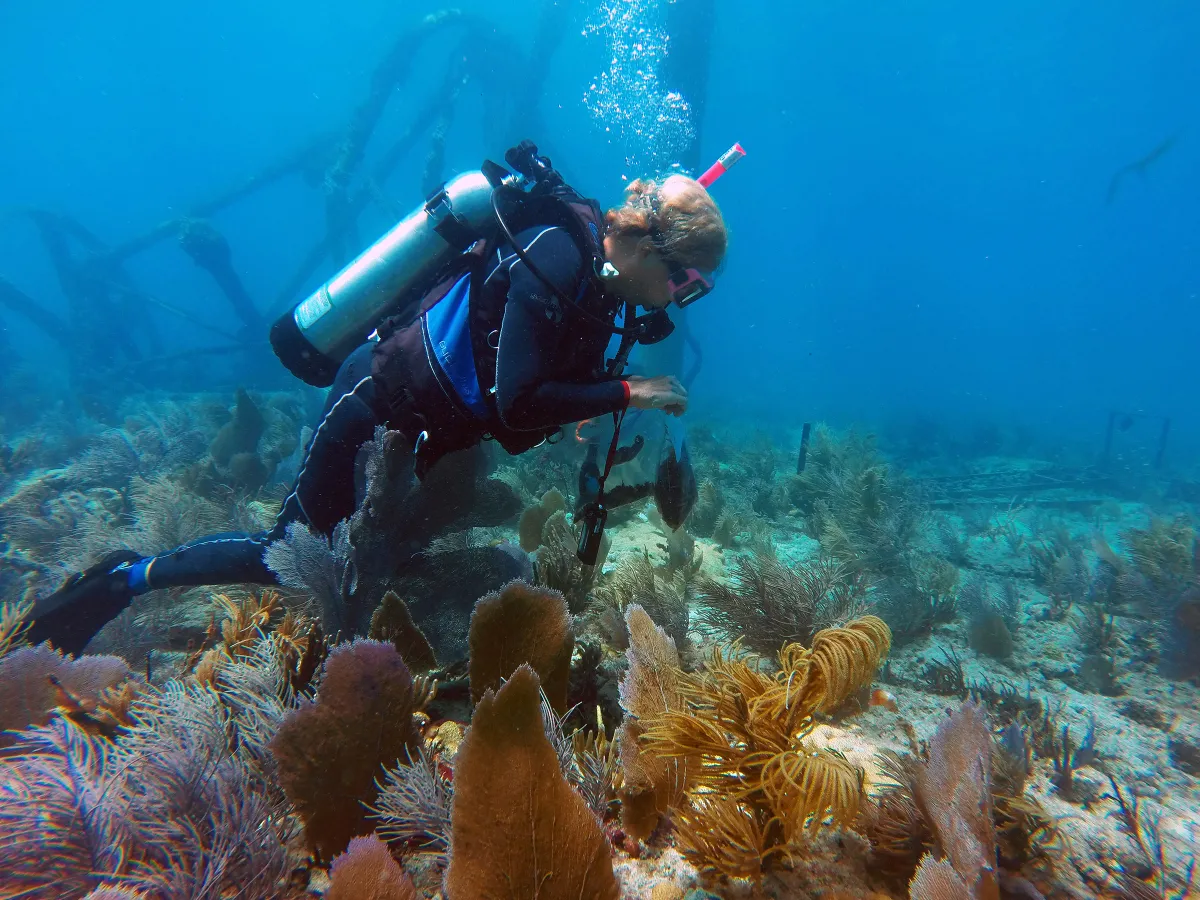
Search
Decrypting defenses
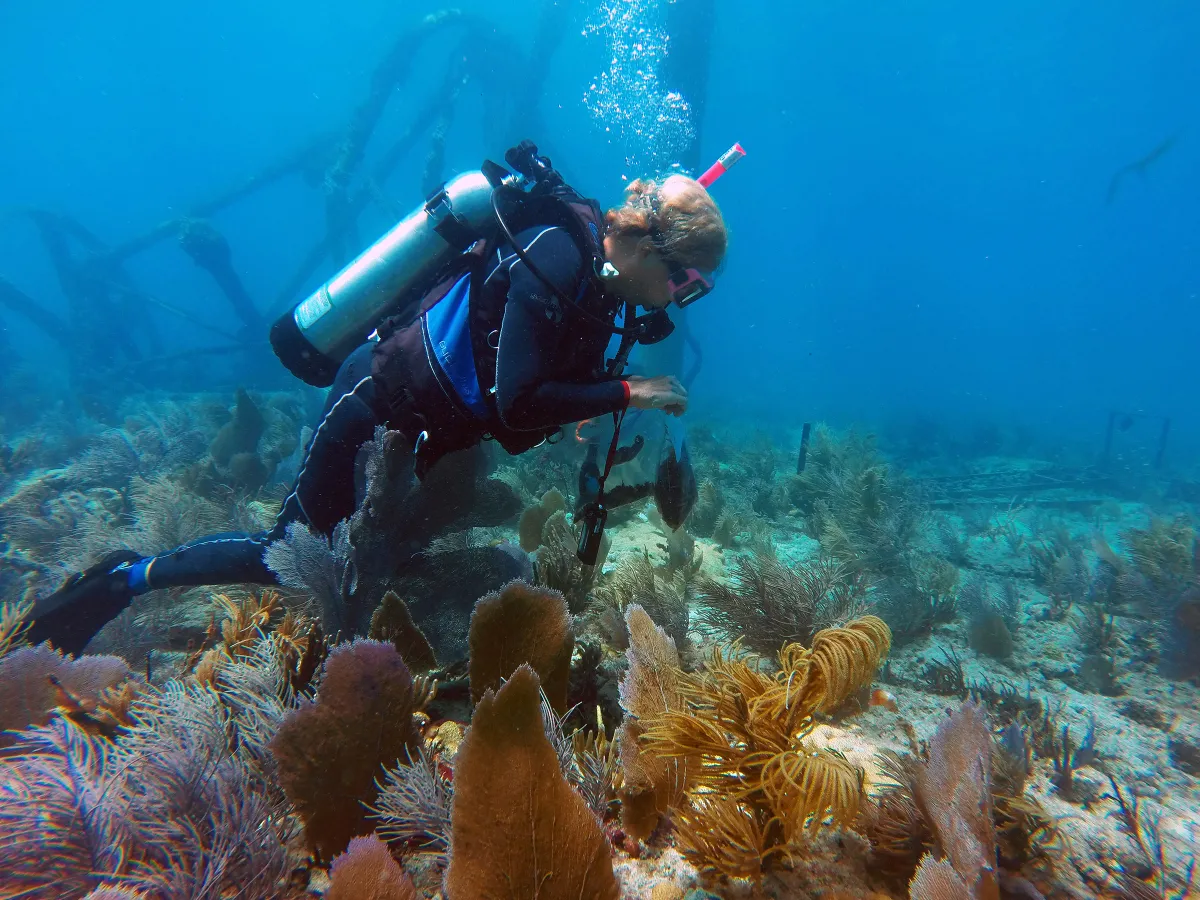
Decrypting defenses
Smithsonian research into cyanobacteria’s powerful chemical compounds yields benefits for humans as well as reef habitats.
By Michelle Z. Donahue
Perhaps more in the ocean than in other environments, the fight for survival is fierce. In that battle, one group of primordial organisms have relied not upon tough armor, nor fearsome teeth or clever wit to survive for more than 3 billion years.
Instead, they’ve employed an equally ancient strategy: poison.
Cyanobacteria, also known as blue-green algae, play hugely important roles in the world’s oceans as well as freshwater and on land. They contribute to the oxygen originating from Earth’s oceans, which produce anywhere from 50 to 85 percent of the world’s oxygen supply. In plants, they’re what make leaves green – the chloroplasts inside plant cells are of cyanobacterial origin. Used to produce nitrogen biofertilizers, of interest for biofuel use, and even as alternatives to conventional antibiotics, cyanobacteria are also found in human dietary supplements, including vitamins and spirulina tablets.
However, many species produce harmful toxins, including those that cause the well-known and harmful algal blooms that occur in lakes, estuaries and other aquatic environments. And although these toxins are an innate defense mechanism, it’s the dose that makes the difference between poison and remedy: used shrewdly, cyanobacteria-derived compounds have shown to be powerful human therapeutics.
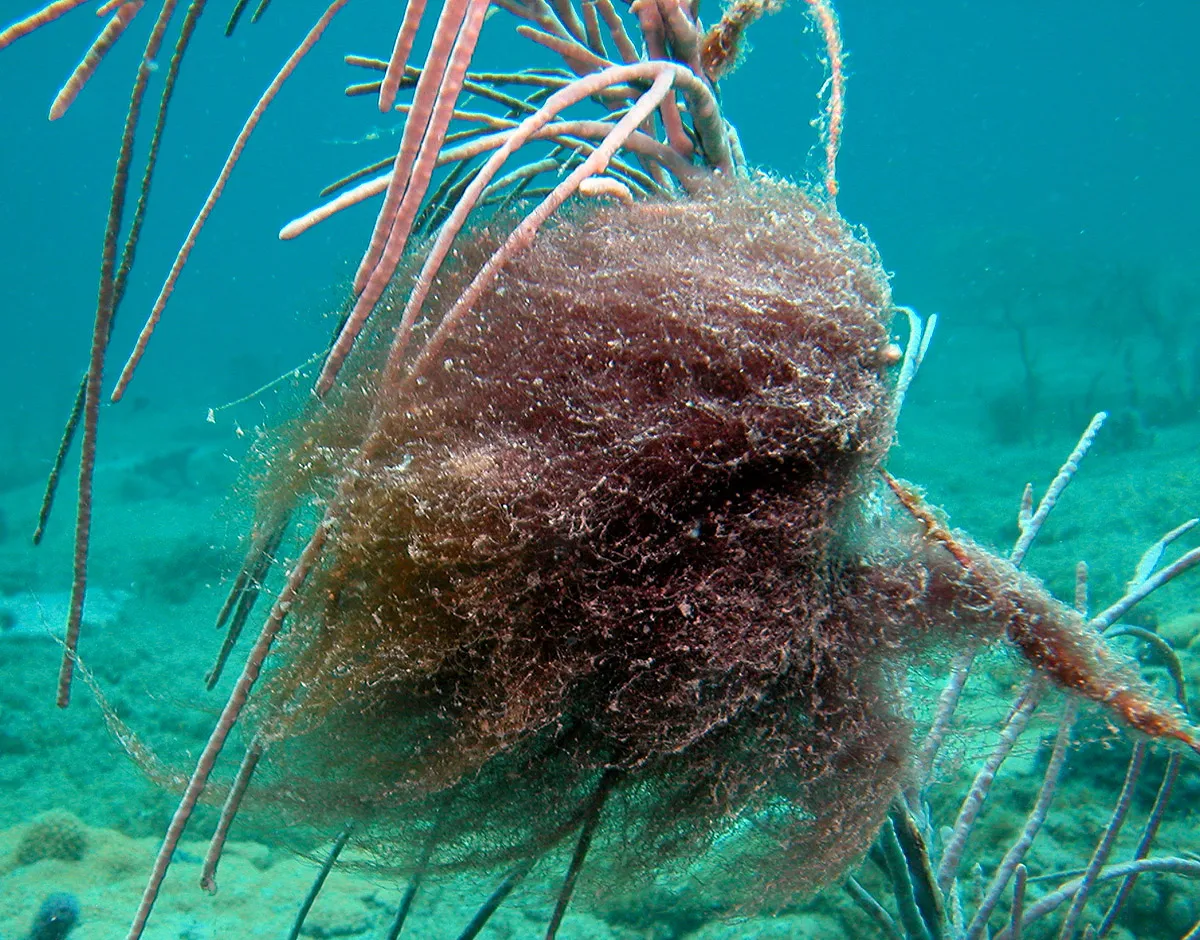
SMS Director Dr. Valerie Paul has been studying cyanobacteria on tropical reefs since the 1990s. Part of her career-long investigations into chemical signals produced by marine organisms, Paul studies how those signals influence the ecology and behavior of other organisms. With ongoing funding from the National Institutes of Health, Paul and Dr. Hendrik Luesch, a longtime collaborator at the University of Florida, have been looking specifically at cyanobacteria whose cytotoxic, or cell-killing, tendencies might make them effective at targeting a particular class of cells: cancer.
“I started noticing cyanobacteria blooming on reefs when I was working at the University of Guam Marine Laboratory,” Paul said. They piqued a deep curiosity—and working with experts at the University of Hawaii, where she met Luesch, a human-application dimension emerged from the reef studies. “I looked at both the chemical ecology of these blooms but also began to work on the biomedical activity of cyanobacterial natural products,” Paul added.
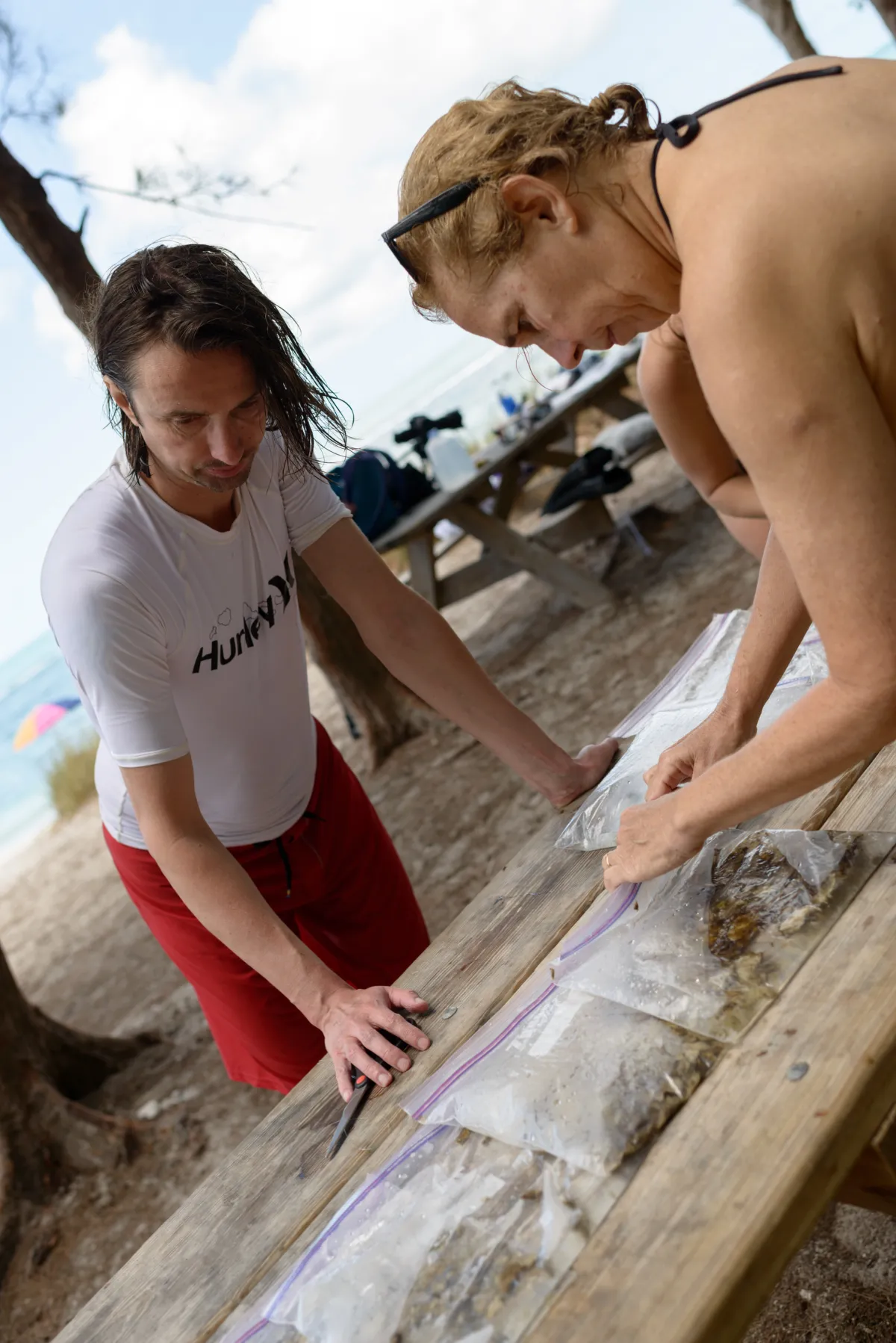
Many such chemicals have already been discovered – to date, around 30,000 marine natural products have been detected, analyzed, and named. Paul and Luesch have contributed to the discovery of over 180 of these.
Despite their potential, few have strong enough effects to warrant further study. Many compounds have also been abandoned as therapeutics not long after discovery for being too broadly toxic to cells, or because they are too difficult to test or produce. Another roadblock is the length of time it can take to go from discovery to drug; the process can take a decade or longer.
However, new techniques and technology are enabling researchers like Luesch and Paul to take a closer look at these cyanobacterial molecules: at how they might be made into medicines, used as biomedical probes to learn about cell and cancer biology, and how cyanobacteria themselves affect other organisms in their natural environments.
In the Wild
Guam’s Apra Harbor is a 20-minute drive to the opposite side of the tiny Pacific island from the University of Guam, where Paul spent 17 years as a researcher. On a specimen collection dive in 1991, in the clear aquamarine waters of Fingers Reef on the south side of the harbor, Paul gathered samples from a species of cyanobacteria then known as Lyngbya (now classified as Moorena bouillonii), growing on and under coral.
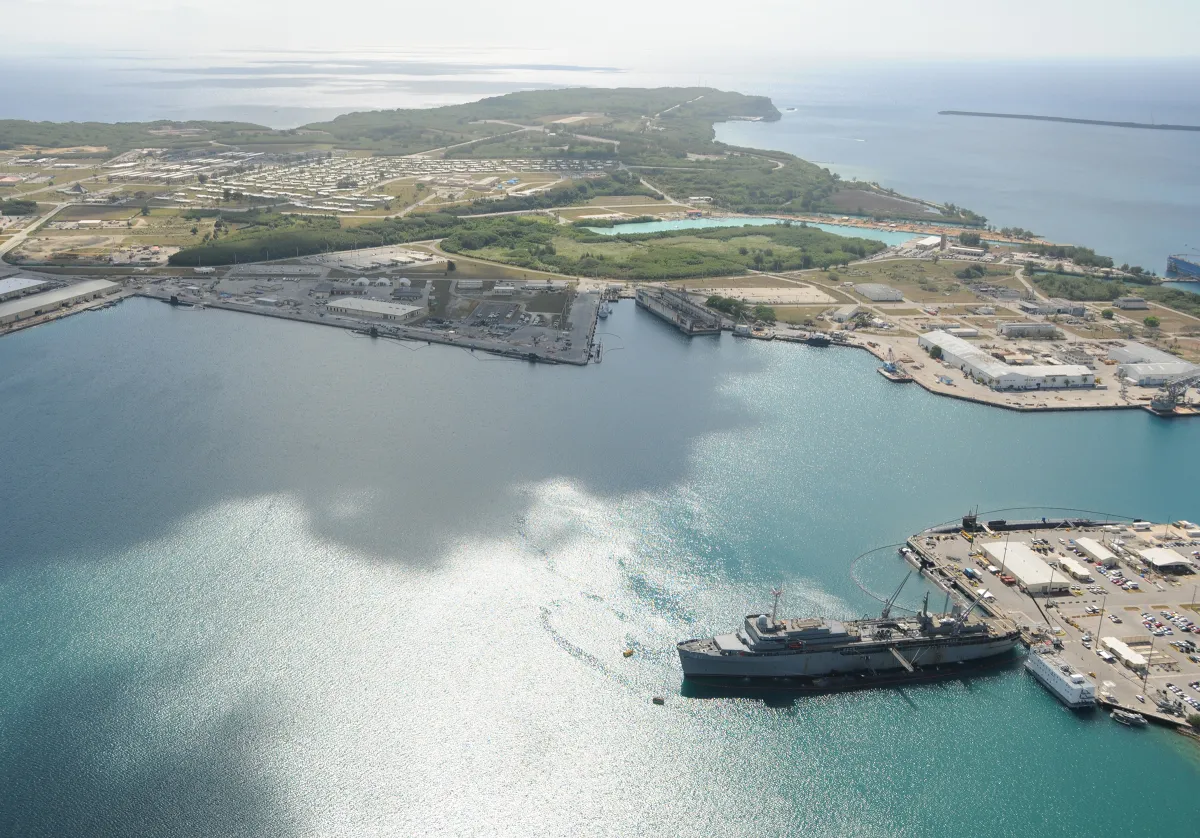
Though cyanobacteria are single-celled organisms, filamentous cyanobacteria can proliferate into very visible colonies. Ocean blooms can cover tens of meters on tropical reefs. And cyanobacteria are ubiquitous, coating many surfaces in a thin scum, or growing in hair-like tufts or as dark puffballs. In Apra Harbor, the cyanobacteria Paul collected looked like tassels of red hair.
“Usually the most disgusting and slimiest organisms are the cyanobacteria,” Luesch said. “But Val has an eye, an instinct, and the expertise to identify these cyanobacteria in the water and the habitats where they reside.”
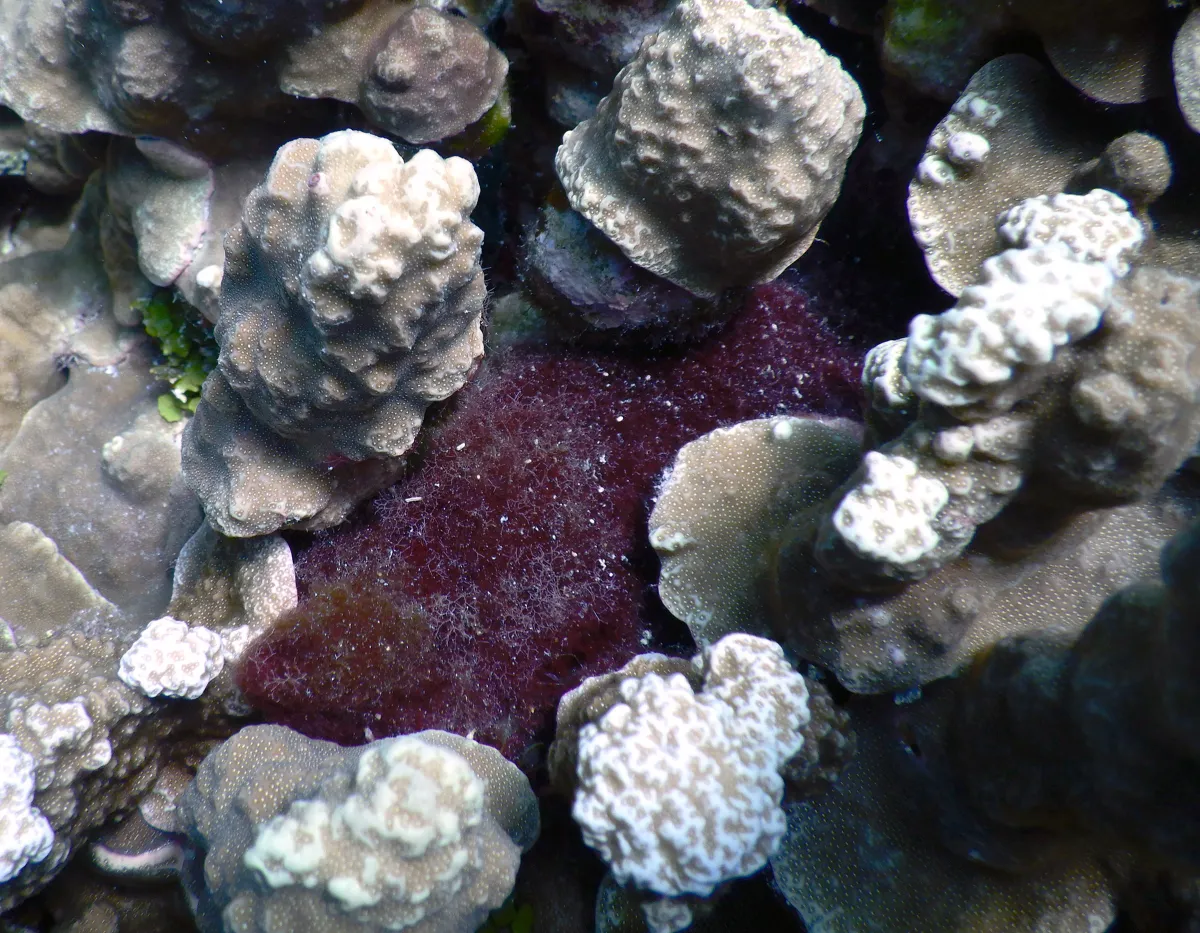
While searching for specimens to collect for research, one clue for Paul are areas where high numbers of herbivorous fish species tend to congregate—and what these grazers avoid eating. “That’s a pretty good indication that what’s left is producing some kind of chemical or physical protection—or sometimes both,” Paul said.
Some animals, like sea slugs, do eat certain types of cyanobacteria in spite of their toxic tendencies—and in fact, sea hares were once intensively researched as a source of marine drugs. That was before Paul, Luesch and their collaborator, University of Hawaii chemist Dr. Richard Moore, discovered that cyanobacteria were the origins of cell-killing compounds known as dolastatins found in the tissues of the Indian Ocean sea hare (Dolabella auricularia). Pinpointing cyanobacteria as the source of the interesting toxins streamlined the process of studying them.
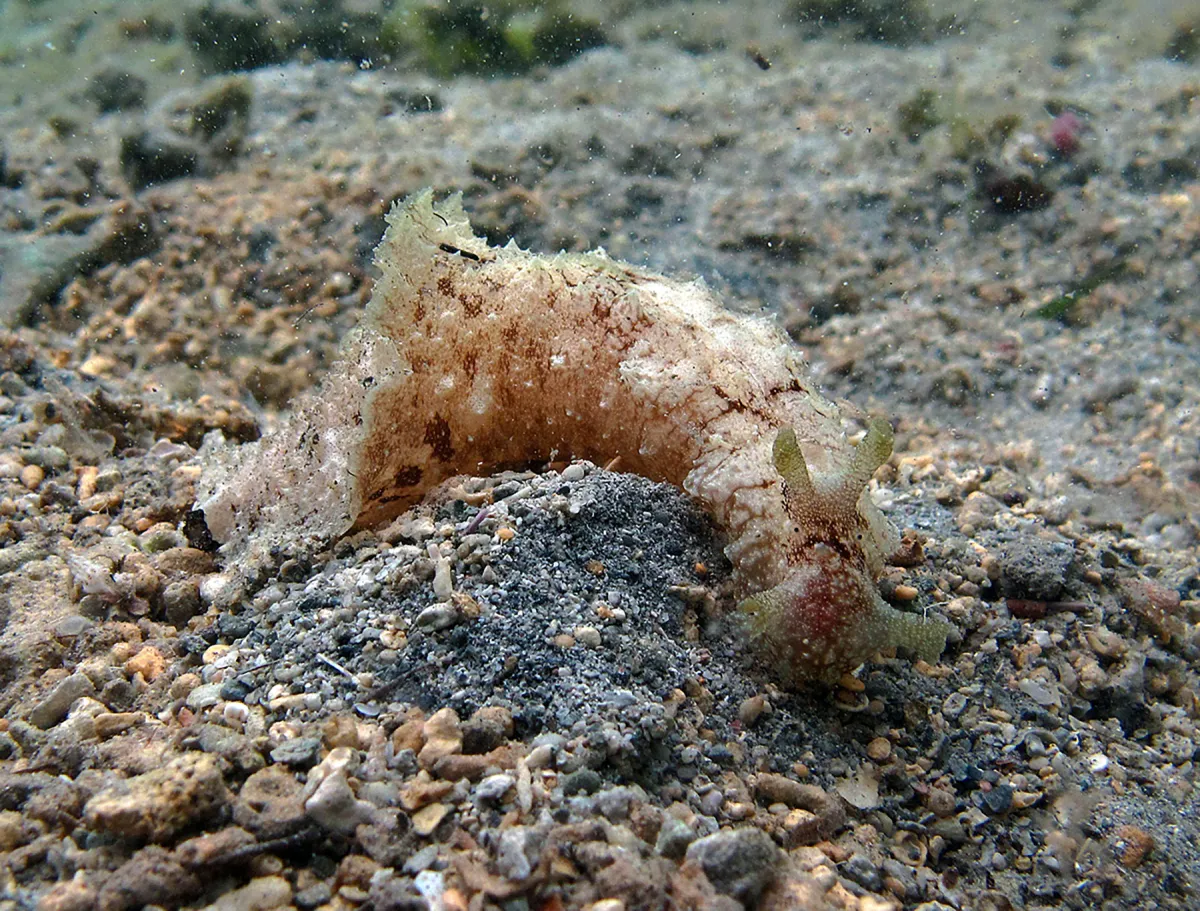
“There’s this evolutionary biochemical warfare going on: organisms producing elaborate compounds to protect themselves, and herbivores evolving detoxification mechanisms to be able to handle them,” Paul added.
The role of cyanobacteria in coral reef ecosystems is still an evolving area of research. While they’re beneficial as a source of nitrogen, some species can bore into rock and coral skeletons, damaging them. When they grow into thick mats directly on corals, they can smother and even poison the coral beneath. Their potent chemicals may inhibit coral larvae from settling on certain areas of a reef. And over the last 40 years, researchers have documented an increase of cyanobacterial growth on reefs, so a more nuanced understanding of their interplay with other reef organisms becomes more important given the other current threats to reefs.
But even the identity of many of these cyanobacteria is still unclear or completely unknown—meaning that much remains to be discovered.
“There are very few natural products researchers looking at cyanobacteria, and there’s a huge genetic diversity out there, which translates by definition into chemical diversity,” Luesch said. “They’re not geared for cancer, so we’re repurposing them for treating disease. But if we knew what they were doing out there on the reef, well, that makes our jobs easier.”
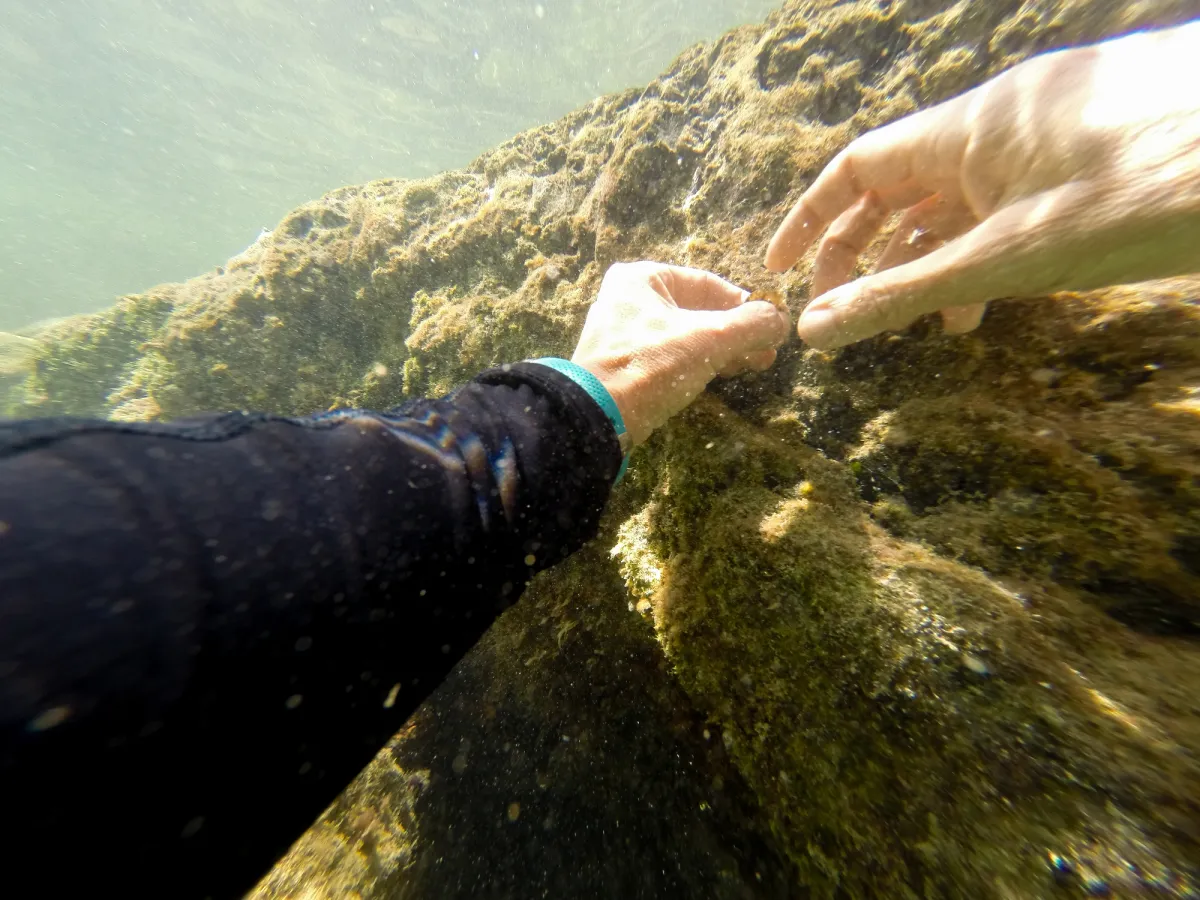
In 2001, 10 years after Paul collected the Moorena bouillonii in Apra Harbor, she would help Luesch define and ultimately name the cytotoxic compound discovered from that sample: apratoxin A. Because they inhibit the formation of new blood vessels, apratoxins are being investigated for many therapeutic uses: against vascularized cancer and for some eye disorders that lead to vision loss, for starters.
Luesch has used this compound as a starting point to develop synthetic drug candidates—through chemical synthesis, natural compounds can be recreated and improved upon, providing the basis for drug development. For instance, a synthetic apratoxin has shown promise for treating pancreatic cancer, one of the deadliest forms of the disease.
Into the Lab
You may not realize it, but your morning coffee is a daily exercise in chemistry. Pouring boiling water over the grounds doesn’t just create a warm, invigorating morning experience; it extracts the chemical compounds that give coffee its flavor and character: caffeine, antioxidants, and other bioactive molecules.
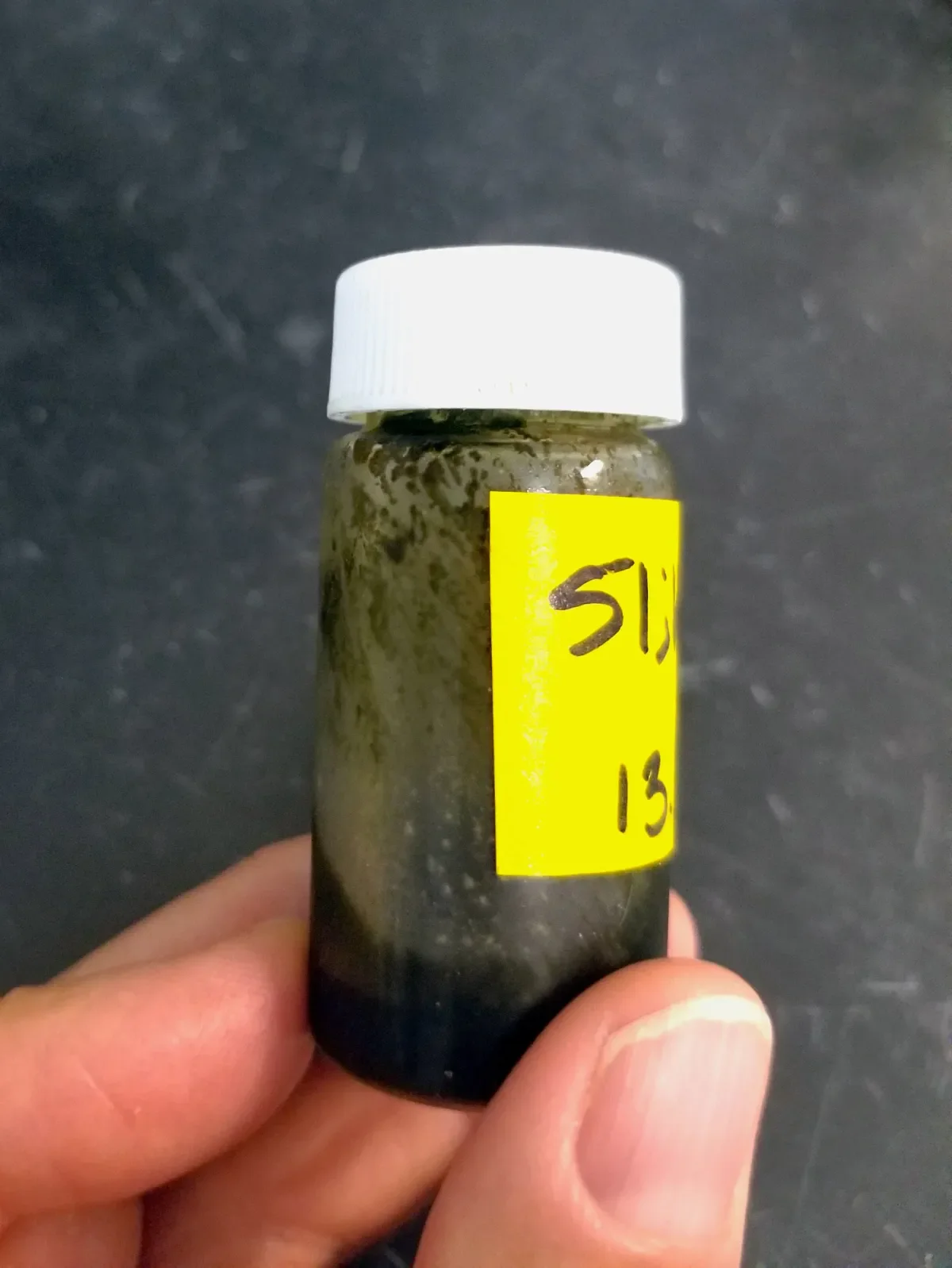
Extracting active compounds from cyanobacteria involves a similar—if more complicated—process. At SMS, chemist Dr. Sarath Gunasekera works constantly at sleuthing out the molecules that make up the extracts, and the chemical structures of those molecules.
Gunasekera has also long been involved in discovering new potential drugs from marine organisms –including discodermolide, isolated from the deep-sea sponge Discodermia dissoluta.
The process begins by dissolving a sample of cyanobacteria, which ultimately results in a dark green, often sticky goo. Sometimes a bit of the entire crude extract goes to Luesch’s lab, which tests its effects on lab-grown cancer cells; if the extract does kill those cells, ideally leaving healthy cells alive, then Gunasekera and the team in the Luesch lab work to isolate the compound(s) responsible for that activity.
When a compound has been purified – that is, only one molecule is present in a sample – the next step is to determine the structure and shape of the molecule. Just as the metal prongs of a plug fit into an electrical socket to allow electrons to flow, so a molecule’s shape and other properties determine how it interacts within a cell (its mechanism of action).
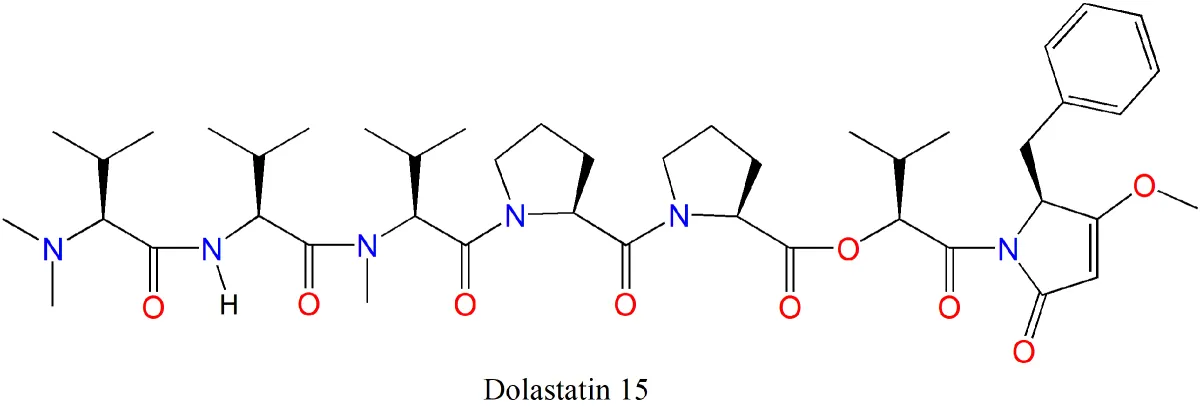
“Sometimes structural features can give away what the molecule may target,” Luesch said. For instance, Luesch’s work on largazole—named for Key Largo, where Paul originally collected the cyanobacterium that produces it in 2003—revealed it was similar to a previously discovered molecule. That made it much more straightforward to work towards incorporating largazole into potential therapies for colon cancer, breast and brain cancer, and even bone disorders.
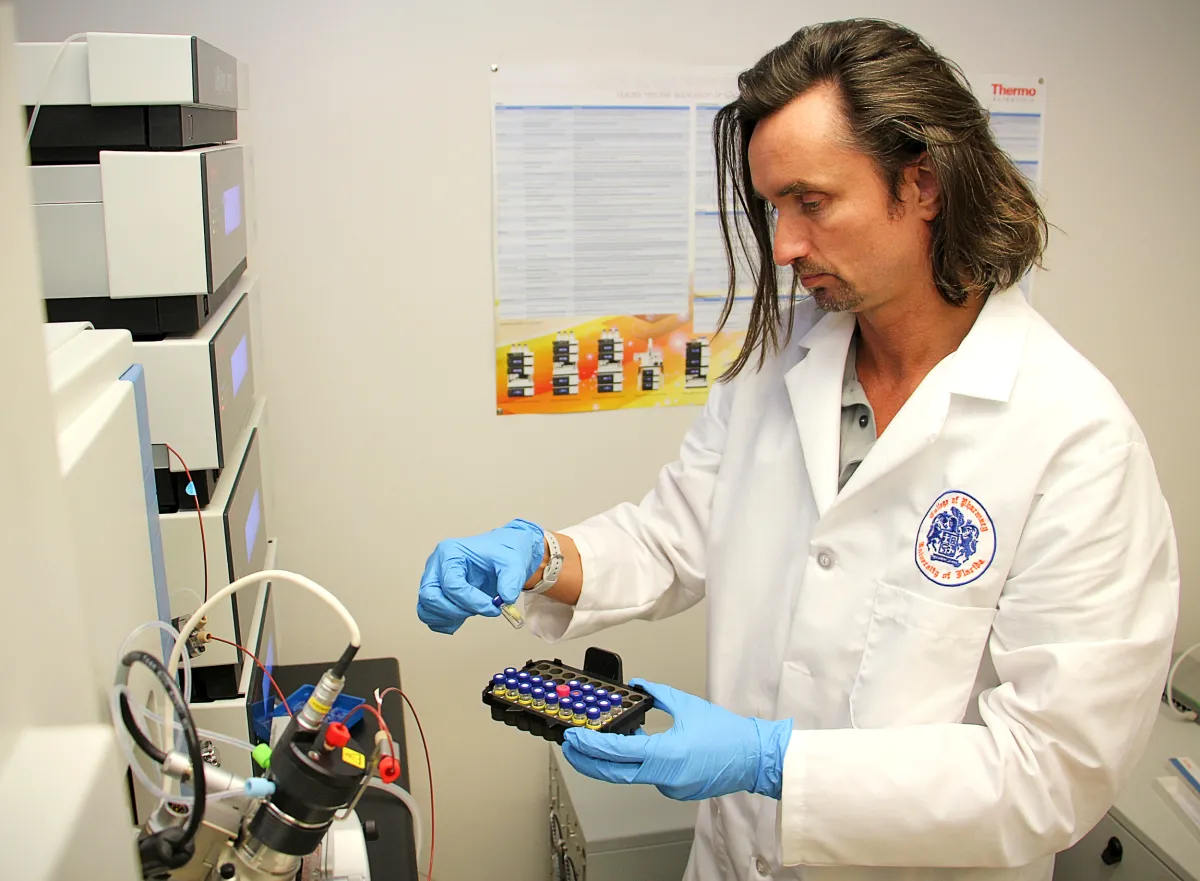
But in molecules where the chemical structure has never been cataloged before—it’s new to science—“that means there’s a chance that it has a novel way of interacting with a cellular target, or that it has a novel target and mechanism of action,” added Luesch.
Advances in drug delivery technology have also made it possible to revisit cyanobacterial compounds previously determined to be too toxic for practical use. Dolastatin 10 is one such, first discovered in sea hares, then directly isolated from cyanobacteria by Luesch and Paul.
In recent years, other research teams have found that by binding a synthetic compound (derived from dolastatin 10) to an antibody, the drug can be targeted directly at cancer cells, leaving nearby healthy cells unharmed. This “antibody-drug conjugate” breathed new life into uses for dolastatins. In 2011, it was approved by the Food and Drug Administration for the treatment of Hodgkins lymphoma; in 2019, by switching the antibody, it was approved for treatments for b-cell lymphoma; the same year, yet another antibody change earned it further approval for treatment of refractory bladder cancer.
“By coupling these compounds with innovative delivery methods, it’s a chance to resurrect a lot of natural products that haven’t been considered,” Luesch said. “The ultimate goal is to see if these compounds can be useful.”
Next New Discoveries
Paul and Luesch will continue investigating extracts from marine cyanobacteria with a five-year renewal of their cancer research grant from the NIH’s National Cancer Institute. The work has been “highly productive,” Luesch said.
“I’m always hopeful and confident that the next interesting compound is just around the corner,” he added. “Given our success rate, it’s just a matter of time. We’re just a few extracts away from something better.”
Unlike an inventor who is able to see others using his or her creations, Luesch and Paul rarely have opportunities to directly observe the impact of their work, primarily due to the prolonged process of drug development after discovery. However, Luesch recalled a chance encounter with a physician colleague at the University of Florida who connected Luesch’s name with his work on dolastatin 10. The doctor told Luesch that he was treating patients in his clinic with dolastatin-based drugs, and expressed pleasure at closing the circle between the treatment and its early origins.
“That was surprising and encouraging,” Luesch said.
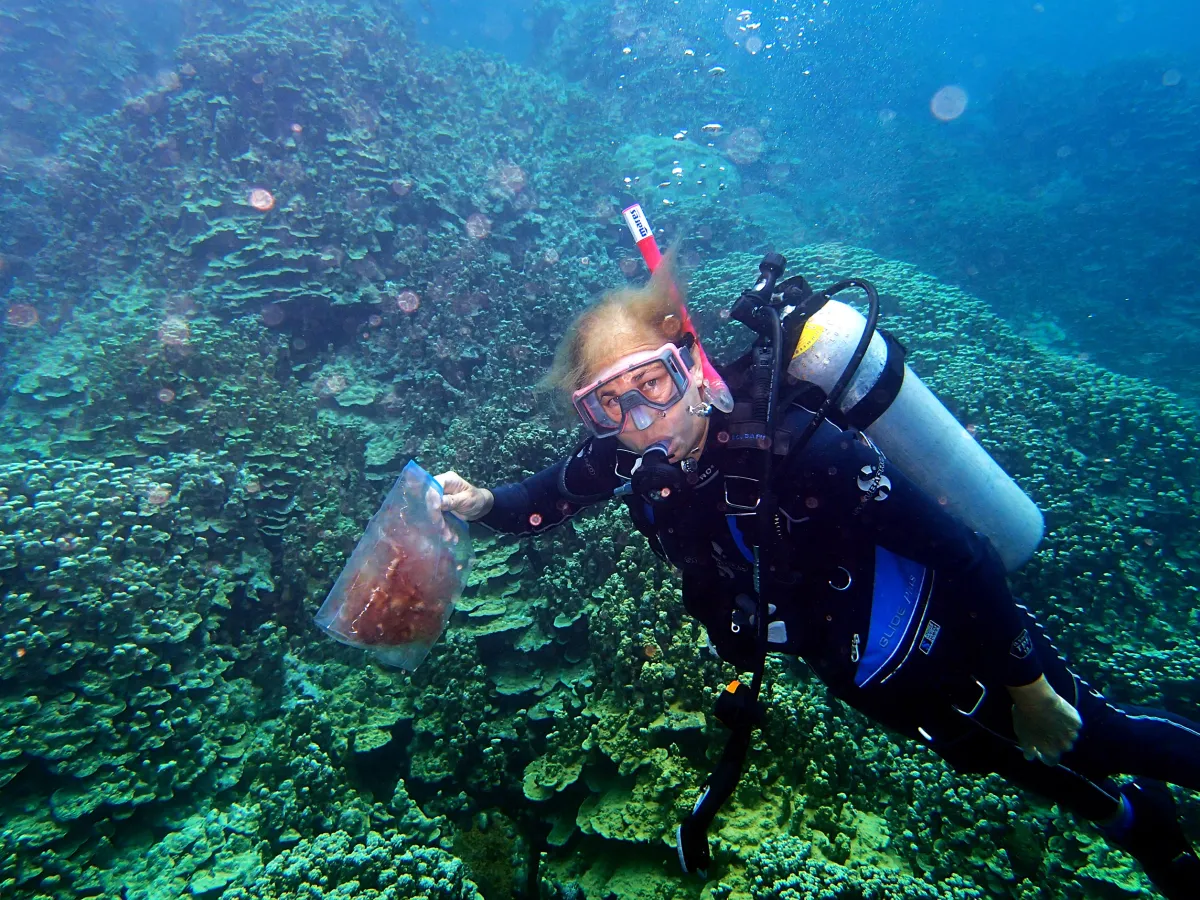
For Paul, the basic research endeavor that is learning more about cyanobacterial natural products—how these compounds function in nature, as well as how they function in human cells—motivates her, and her curiosity is infectious. “The cool thing is…” she often quips when referring to several different prongs of her research -- toxins from flatworms, chemical extracts from nudibranchs and the sponges they eat, the structure and function of dolastatin 15.
“There are a lot of different ways this work can advance knowledge,” Paul said. “Meshing organic chemistry with natural products, cell biology with reef ecology – you name it. There are many ways this fundamental knowledge can be utilized.”
August 2020